The cell cycle is a four-stage process in which the cell increases in size (gap 1, or G1, stage), copies its DNA (synthesis, or S, stage), prepares to divide (gap 2, or G2, stage), and divides (mitosis, or M, stage). The stages G1, S, and G2 make up interphase, which accounts for the span between cell divisions.
A cell cycle is a series of events that takes place in a cell as it grows and divides. A cell spends most of its time in what is called interphase, and during this time it grows, replicates its chromosomes, and prepares for cell division. The cell then leaves interphase, undergoes mitosis, and completes its division. The cell cycle is the replication and reproduction of cells, whether in eukaryotes or prokaryotes.
It is important to organisms in different ways, but overall it allows them to survive.
For prokaryotes, the cell cycle, called Binary Fission, allows for them to live on by dividing into two new daughter cells.
For eukaryotes, consider an animal such as a cat, if a cat were to have a severe injury, it would need the cell cycle to replicate cells to replace those that were damaged in the injury and hence needs the cell cycle to heal itself.
Zygotes also depend on the cell cycle to form its many cells in order to produce a baby organism at the end of its process.
Plants require the cell cycle to grow and provide life for every other organism on earth. and therefore the cell cycle is highly important to organisms as if it were not present or if it were to stop suddenly, life would completely end.
The cell cycle is an ordered series of events involving cell growth and cell division that produces two new daughter cells. Cells on the path to cell division proceed through a series of precisely timed and carefully regulated stages of growth, DNA replication, and division that produces two identical (clone) cells. The cell cycle has two major phases: interphase and the mitotic phase (Figure 1). During interphase, the cell grows and DNA is replicated. During the mitotic phase, the replicated DNA and cytoplasmic contents are separated, and the cell divides.
Interphase
During interphase, the cell undergoes normal growth processes while also preparing for cell division. In order for a cell to move from interphase into the mitotic phase, many internal and external conditions must be met. The three stages of interphase are called G1, S, and G2.
G1 Phase (First Gap)
The first stage of interphase is called the G1 phase (first gap) because, from a microscopic aspect, little change is visible. However, during the G1 stage, the cell is quite active at the biochemical level. The cell is accumulating the building blocks of chromosomal DNA and the associated proteins as well as accumulating sufficient energy reserves to complete the task of replicating each chromosome in the nucleus.
S Phase (Synthesis of DNA)
Throughout interphase, nuclear DNA remains in a semi-condensed chromatin configuration. In the S phase, DNA replication can proceed through the mechanisms that result in the formation of identical pairs of DNA molecules—sister chromatids—that are firmly attached to the centromeric region. The centrosome is duplicated during the S phase. The two centrosomes will give rise to the mitotic spindle, the apparatus that orchestrates the movement of chromosomes during mitosis. At the center of each animal cell, the centrosomes of animal cells are associated with a pair of rod-like objects, the centrioles, which are at right angles to each other. Centrioles help organize cell division. Centrioles are not present in the centrosomes of other eukaryotic species, such as plants and most fungi.
G2 Phase (Second Gap)
In the G2 phase, the cell replenishes its energy stores and synthesizes proteins necessary for chromosome manipulation. Some cell organelles are duplicated, and the cytoskeleton is dismantled to provide resources for the mitotic phase. There may be additional cell growth during G2. The final preparations for the mitotic phase must be completed before the cell is able to enter the first stage of mitosis.
The Mitotic Phase
The mitotic phase is a multistep process during which the duplicated chromosomes are aligned, separated, and move into two new, identical daughter cells. The first portion of the mitotic phase is called karyokinesis, or nuclear division. The second portion of the mitotic phase, called cytokinesis, is the physical separation of the cytoplasmic components into the two daughter cells.
Karyokinesis (Mitosis)
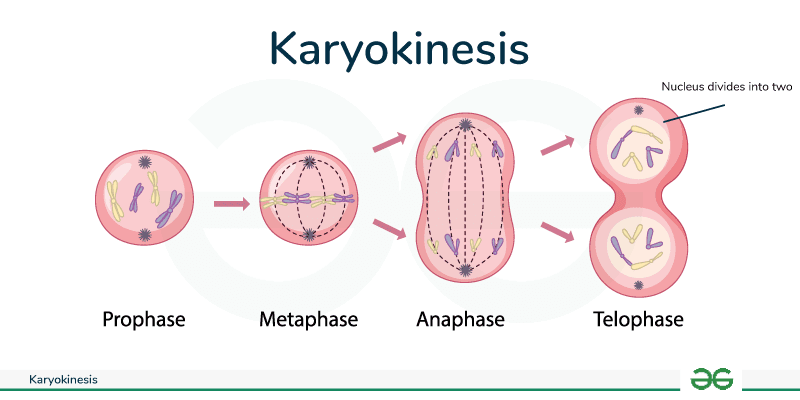
Karyokinesis, also known as mitosis, is divided into a series of phases—prophase, prometaphase, metaphase, anaphase, and telophase—that result in the division of the cell nucleus (Figure 2). Karyokinesis is also called mitosis.
During prophase, the “first phase,” the nuclear envelope starts to dissociate into small vesicles and the membranous organelles (such as the Golgi complex or Golgi apparatus, and endoplasmic reticulum), fragment and disperse toward the periphery of the cell. The nucleolus disappears (disperses). The centrosomes begin to move to opposite poles of the cell. Microtubules that will form the mitotic spindle extend between the centrosomes, pushing them farther apart as the microtubule fibers lengthen. The sister chromatids begin to coil more tightly with the aid of condensing proteins and become visible under a light microscope.
During prometaphase, the “first change phase,” many processes that were begun in prophase continues to advance. The remnants of the nuclear envelope fragment. The mitotic spindle continues to develop as more microtubules assemble and stretch across the length of the former nuclear area. Chromosomes become more condensed and discrete. Each sister chromatid develops a protein structure called a kinetochore in the centromeric region. The proteins of the kinetochore attract and bind mitotic spindle microtubules. As the spindle microtubules extend from the centrosomes, some of these microtubules come into contact with and firmly bind to the kinetochores. Once a mitotic fiber attaches to a chromosome, the chromosome will be oriented until the kinetochores of sister chromatids face the opposite poles. Eventually, the entire sister chromatids will be attached via their kinetochores to microtubules from opposing poles. Spindle microtubules that do not engage the chromosomes are called polar microtubules. These microtubules overlap each other midway between the two poles and contribute to cell elongation. Astral microtubules are located near the poles, aid in spindle orientation, and are required for the regulation of mitosis.
During metaphase, the “change phase,” all the chromosomes are aligned in a plane called the metaphase plate, or the equatorial plane, midway between the two poles of the cell. The sister chromatids are still tightly attached to each other by cohesin proteins. At this time, the chromosomes are maximally condensed.
During anaphase, the “upward phase,” the cohesin proteins degrade, and the sister chromatids separate at the centromere. Each chromatid, now called a chromosome, is pulled rapidly toward the centrosome to which its microtubule is attached. The cell becomes visibly elongated (oval shaped) as the polar microtubules slide against each other at the metaphase plate where they overlap.
During telophase, the “distance phase,” the chromosomes reach the opposite poles and begin to decondense (unravel), relaxing into a chromatin configuration. The mitotic spindles are depolymerized into tubulin monomers that will be used to assemble cytoskeletal components for each daughter cell. Nuclear envelopes form around the chromosomes, and nucleosomes appear within the nuclear area.
Cytokinesis
Cytokinesis, or “cell motion,” is the second main stage of the mitotic phase during which cell division is completed via the physical separation of the cytoplasmic components into two daughter cells. Division is not complete until the cell components have been apportioned and completely separated into the two daughter cells. Although the stages of mitosis are similar for most eukaryotes, the process of cytokinesis is quite different for eukaryotes that have cell walls, such as plant cells.
In cells such as animal cells that lack cell walls, cytokinesis follows the onset of anaphase. A contractile ring composed of actin filaments forms just inside the plasma membrane at the former metaphase plate. The actin filaments pull the equator of the cell inward, forming a fissure. This fissure, or “crack,” is called the cleavage furrow. The furrow deepens as the actin ring contracts, and eventually the membrane is cleavednj in two.
In plant cells, a new cell wall must form between the daughter cells. During interphase, the Golgi apparatus accumulates enzymes, structural proteins, and glucose molecules prior to breaking into vesicles and dispersing throughout the dividing cell. During telophase, these Golgi vesicles are transported on microtubules to form a phragmoplast (a vesicular structure) at the metaphase plate. There, the vesicles fuse and coalesce from the center toward the cell walls; this structure is called a cell plate. As more vesicles fuse, the cell plate enlarges until it merges with the cell walls at the periphery of the cell. Enzymes use the glucose that has accumulated between the membrane layers to build a new cell wall. The Golgi membranes become parts of the plasma membrane on either side of the new cell wall.
G0 Phase
Not all cells adhere to the classic cell cycle pattern in which a newly formed daughter cell immediately enters the preparatory phases of interphase, closely followed by the mitotic phase. Cells in G0 phase are not actively preparing to divide. The cell is in a quiescent (inactive) stage that occurs when cells exit the cell cycle. Some cells enter G0 temporarily until an external signal triggers the onset of G1. Other cells that never or rarely divide, such as mature cardiac muscle and nerve cells, remain in G0 permanently.
Chromosome Number
The number of chromosomes present in each somatic cell, which is constant for any one species of plant or animal. In the reproductive cells this number is halved, In humans, each cell normally contains 23 pairs of chromosomes, for a total of 46. Twenty-two of these pairs, called autosomes, look the same in both males and females. The 23rd pair, the sex chromosomes, differ between males and females. A change in the number of chromosomes can cause problems with growth, development, and function of the body’s systems.
Scientists have numbered the chromosome pairs from 1 to 22, with the 23rd pair labeled as X or Y, depending on the structure. The first 22 pairs of chromosomes are called autosomes. The 23rd pair of chromosomes are known as the sex chromosomes, because they decide if you will be born male or female.
In a given species, chromosomes can be identified by their number, size, centromere position, and banding pattern. In a human karyotype, autosomes or “body chromosomes” (all of the non–sex chromosomes) are generally organized in approximate order of size from largest (chromosome 1) to smallest (chromosome 22).
Types of Chromosome
Among many organisms that have separate sexes, there are two basic types of chromosomes: sex chromosomes and autosomes. Autosomes control the inheritance of all the characteristics except the sex-linked ones, which are controlled by the sex chromosomes. Humans have 22 pairs of autosomes and one pair of sex chromosomes.
Humans have 46 chromosomes in each diploid cell. Among those, there are two sex-determining chromosomes, and 22 pairs of autosomal, or non-sex, chromosomes. The total number of chromosomes in diploid cells is described as 2n, which is twice the number of chromosomes in a haploid cell (n).
Chromosomes: morphology, structure
Chromosomes were first seen by Hofmeister (1848) in the pollen mother cells of Tradescantia in the form of darkly stained bodies. The term chromosome (Gr: chrom=colour; soma=body) was used by Waldeyer (1888) to designate their great affinity to basic dyes.
Their functional significance was described by IV.S. Sutton (1900) when he traced parallelism between segregation of chromosomes during meiosis and transmission of hereditary factors during gametoeenesis. General reviews on the morphology of chromosomes have been published by Heitz (1935), Kuwada (1939), Geitler (1940) and Kaufmann (1948).
Chromosomes are the most significant components of the cell, particularly they are apparent during mitosis and meiosis. Their presence was demonstrated long before they were named “chromosomes” by Waldeyer in 1888.
A chromosome can be considered as a nuclear component having special organization, individuality and function. It is capable of self-reproduction while maintaining its morphologic and physiologic properties through successive cell divisions.
Morphology:

The morphology of chromosome can be best studies at the metaphase or anaphase of mitosis when they are present as definite organelles, being most condensed or coild.
Number:
The number of chromosomes in a given species is usually constant containing diploid number (2n) of chromosomes in their somatic cells and haploid (gametic or reduced) number (n) of chromosomes in their sex cells (sperms and ova). The number of chromosomes is variable from one to several hundred among different species
For example, in Ascaris megalocephala it is 2, while in certain protozoans (Aggreta), there are more than 300 chromosomes, in Paramecium 30 to 40, in radiolarians as many as 1600, in Hydra vulgaris 32, Musca domestica 12, Rana esculenta 26, Columba livia 80, Oryctolagus cuniculus 44, Gorilla gorilla 48 and Homo sapiens (man) 46.
The chromosome numbers are also helpful for taxonomy. In the angiosperms the most frequent haploid number is 12 and members of this group have a range from 3 to 16. Similarly, in fungi, haploid number ranges from 3 to 8.
In primates this haploid number is from 16 to 30. This haploid set of chromosomes present in the nucleus of gametes in while in a diploid cell there will be two genomes. The diploid cells are the somatic cells in body. The diploid cells get the diploid set of chromosomes by the union of the haploid male and female gametes in the sexual reproduction.